In light of the current energy crisis and environmental pollution, the storage, conversion, and utilization of clean energy have become the focus of current research, with electrocatalytic technology playing a crucial role in this regard.
Localized Surface Plasmon Resonance (LSPR) specifically refers to the collective resonance phenomenon of conduction electrons in coinage metal or heavily doped semiconductor nanostructures under the excitation of incident light at a specific wavelength.
It can efficiently control the reaction process under more moderate conditions and has been widely used in electrocatalytic systems such as organic small molecule oxidation, water splitting, and oxygen reduction.
Research has shown that the photoelectron effect and photothermal effect generated by plasmons during relaxation are the main mechanisms for multidimensional regulation of the activity and selectivity of electrocatalytic reactions.
However, how to distinguish and quantitatively reveal the relative contributions of the two effects in the actual electrocatalytic reaction process is still an unsolved key issue in the field, and it is also a bottleneck restricting the further development of this field.Professor Cai Wenbin from Fudan University and his team hope to deepen the understanding of key issues in specific fields, using a combination of photoelectrochemical experiments and in-situ attenuated total reflection surface-enhanced infrared spectroscopy (ATR-SEIRAS, Attenuated Total Reflection-Surface Enhanced Infrared Absorption Spectroscopy) to deeply analyze the mechanism of LSPR-mediated electrocatalytic systems at both the macroscopic and molecular levels.
Advertisement
According to the introduction, most previous studies have focused on the design and modification of high-activity catalytic materials, but there is a lack of in-depth understanding of the mechanism of plasmon and the electrochemical reactions it mediates.
This can be perfectly combined with the research characteristics of their group, that is, to use the advantages of the group in in-situ infrared spectroscopy.
They hope to use visible light to excite the reaction and mid-infrared light to detect the reaction, to deeply clarify the mechanism of LSPR-mediated electrocatalytic reactions.
Specifically, their work focuses on a scientific issue that is currently of great concern in the field but has not yet been fully resolved: that is, the quantitative decoupling of photoelectron effects and photothermal effects in actual electrocatalytic systems.In their research, they selected the model catalytic system of CO2 reduction to CO on Ag nanoparticles for CO2RR (carbon dioxide reduction reaction). Ag nanoparticles can serve both as a typical plasmonic metal and as a metal catalyst for CO2RR, with simple synthesis methods and well-defined composition and structure.
The reduction product CO on Ag can be used as fuel or chemical raw material. Hydrocarbons can be generated through Fischer-Tropsch synthesis or further electrochemical reduction reactions, making it a highly promising catalytic strategy.
At the same time, the target reaction and side reactions of CO2RR to produce CO are clear, which is beneficial for modeling studies on the mechanism of LSPR (localized surface plasmon resonance) effects.
According to existing literature, the research team synthesized Ag nanoparticles of the required size, ensuring good reproducibility, uniform size, and good optical properties.
At the same time, they conducted selective tests on the photocatalytic CO2RR activity and product selectivity of the catalyst to ensure the reliability of the experimental results.In fact, many researchers have contributed to the emerging field of LSPR-mediated electrocatalysis, but the study of the mechanism of plasmonic effects under actual catalytic systems has always been a weak point in the field.
Previously, people mostly used the perspective of traditional photochemistry to simply explain the mechanism of electrochemical reactions, but often overlooked the impact of different potentials on different LSPR effects, which is exactly the characteristic of the field of LSPR-mediated electrocatalysis.
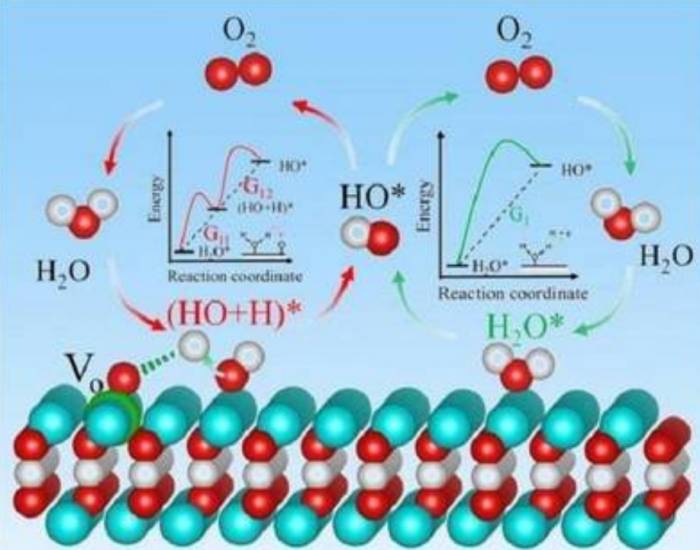
At the beginning of the research, they set the direction on the LSPR-mediated CO2RR system on the CuAg dimer cascade catalyst. They hope to use the LSPR effect of Ag to adjust the activity and selectivity of C2 products on the Cu-based catalyst, and further combine in-situ infrared to study the reaction mechanism.
The design and synthesis of the required morphology of the catalyst has already taken them a certain amount of time. During the electrochemical experiment, they found that the excitation of LSPR has no obvious effect on the reaction activity and selectivity.
Moreover, due to the complexity of the system, it is difficult to reasonably attribute the observed peaks in the infrared, which has led the research to a bottleneck.At this point, by reviewing and summarizing the experiments throughout the entire project period, they found that simply using silver (Ag) nanoparticles could achieve excellent reaction performance, and the preparation method of Ag is more simple and efficient, making it an outstanding model catalyst for mechanism research.
Following this line of thought, they conducted extensive literature research and found breakthroughs and innovation points.
To elaborate on the reaction mechanism of surface plasmon catalysis in detail, the team, based on their previous work [1], ingeniously designed photocurrent experiments under different potentials and different reaction atmospheres (CO2 and Ar), in order to more easily observe the different LSPR effects and their respective impacts on CO2RR for generating CO and the hydrogen evolution side reaction under potential modulation.
The results showed that the photoelectron effect mainly promotes the generation of CO from CO2RR, while the photothermal effect is more conducive to the hydrogen evolution process.
Furthermore, in situ ATR-SEIRAS revealed this rule at the molecular level: LSPR enhances the generation of bridged CO on the surface of Ag nanoparticles, thereby improving the selectivity of the product CO.In addition, combining isotope labeling experiments, they also found that the CO2RR process under LSPR excitation and without LSPR excitation has the same carbonyl-containing C1 reaction intermediates.
Overall, this work not only quantitatively reveals the relative contributions of plasmonic photoelectron effects and photothermal effects under potential modulation to actual electrocatalytic reactions, but also provides a reliable strategy that combines photoelectrochemical methods with ATR-SEIRAS technology to deeply analyze the complex mechanism of plasmonic electrocatalysis.
This provides an important basis for establishing an in-depth correlation between photoelectrocatalytic performance, LSPR mechanism, and interfacial electrochemistry.
For the relevant paper, the reviewer said: "The authors quantitatively decouple the relative contributions of photoelectron effects and photothermal effects in the actual electrocatalytic reaction process, and combine in-situ ATR-SEIRAS technology to understand the reaction process at the molecular level.
The establishment and exploration of this methodology are expected to provide more solid support for deepening the understanding of plasmonic electrochemical related mechanisms."Recently, the relevant paper was published in Angewandte Chemie[2] with the title "Uncovering Photoelectronic and Photothermal Effects in Plasmon-Mediated Electrocatalytic CO2 Reduction."
Wei Yan is the first author, and Associate Professor Zhan Chao from Xiamen University and Professor Cai Wenbin from Fudan University serve as co-corresponding authors.
Since this work mainly focuses on basic theoretical research, the research paradigm constructed can also be extended to other new electrocatalytic systems in the field.
On the one hand, under the guidance of the reaction mechanism, new high-efficiency photoelectrocatalytic materials can be customized through a combination of rational design and combination screening.
For example, by changing the morphology, composition, and structure of the catalyst to regulate its LSPR wavelength, the dominant effect in its catalytic process can be targeted for change, thereby further significantly improving the conversion efficiency and selectivity of the photoelectrocatalytic process.On the other hand, through systematic research methodologies, a multi-faceted, in-depth analysis of the mechanisms of new electrocatalytic reactions can be conducted at both the macroscopic and molecular levels.
These two aspects complement and promote each other, providing feedback that can offer new possibilities for the realization of precise and efficient energy electrocatalytic systems.
In terms of practical applications, the development and modification of non-metallic plasmonic materials, as well as the structural design of photoelectrochemical cells and the regulation of electrolytes, are all very important research directions.
Reducing material costs, improving efficiency, and optimizing product design are key steps in the industrialization of plasmon-mediated electrocatalytic systems, and thus they require full consideration for the future.
The research team looks forward to more progress in this field in practical applications, and all of this is inseparable from an in-depth understanding of its fundamental principles. Therefore, they also hope that the results of this study can promote the industry's application in this field.In the characterization of plasmonic catalysts, there is still much to explore in the future.
Firstly, the team will combine in-situ single-particle characterization or simulation technology to visualize the localized electromagnetic/thermal field during the actual electrocatalytic process.
This technology can observe the dynamic changes of the catalyst in real-time during the reaction process, which helps to deeply understand the plasmonic electrochemical reaction mechanism and is also of significant guiding importance for the design and optimization of plasmonic catalysts.
Secondly, the research group plans to expand the research methodology established in this study to other plasmonic metal catalytic systems, and design more targeted plasmonic catalyst structures under the guidance of the mechanism.
Different metals have different electrical, optical, and catalytic properties. By studying and comparing the role of these metals in plasmonic electrocatalysis, higher catalytic performance may be achieved and new reaction mechanisms may be discovered.It is reported that the main research directions of Cai Wenbin's research group are: surface-enhanced infrared spectroscopy methodology, electrocatalytic oxidation of organic small molecules, oxygen reduction, and electroreduction of carbon dioxide, etc.
Surface-enhanced infrared spectroscopy, with its high sensitivity, high selectivity, and non-destructive advantages, can achieve real-time, in-situ monitoring without the need for labeling and is suitable for a variety of samples. It has been widely applied in fields such as chemistry, physics, biology, and more.
In response to the bottlenecks of this method, they have developed a series of window film electrode deposition techniques, expanding the types of electrodes to include various transition metals, alloys, and nanocatalysts.
Through this, they have designed and improved a variety of composite infrared windows, micro-structured silicon wafer windows, and flow spectroscopy cells, enhancing the detection sensitivity and selectivity of the surface-enhanced infrared spectroscopy method, and expanding the frequency detection range and application scope of this method.
In addition, the combination of internal and external reflection modes of infrared spectroscopy, as well as density functional theory calculations, also provides strong theoretical support for a deeper understanding and interpretation of experimental results.It is also reported that the electrocatalytic oxidation of organic small molecules (such as methanol, formic acid, ethanol, acetaldehyde, etc.) and the self-catalyzed hydrogen production from formic acid are of great significance for the development of efficient and environmentally friendly energy conversion technologies.
The disconnection between traditional electrocatalytic basic research systems and actual catalyst systems, as well as the limitations of research methods in elucidating catalytic mechanisms, has always been a challenge in this field.
To overcome these issues, they have carried out interactive research on catalytic mechanisms and catalytic materials, and deeply studied the electrocatalytic properties and mechanisms of action of organic small molecules.
Combining advanced characterization techniques and theoretical calculations, the research team has revealed the intrinsic laws of electrocatalysis of organic small molecules, which can be used to design efficient and environmentally friendly catalysts.
It is worth mentioning that around 2008, the research team proposed lattice interstitial doping with B as an important strategy to enhance the activity and stability of metal electrocatalysts, which has been verified by several peer research teams in the study of different types of electrocatalytic reactions.In the research direction of oxygen reduction, this team has synthesized a series of platinum-based or palladium-based catalysts using non-precious metal doping (boron doping) and alloying (cobalt element), which is of great significance for improving the performance of fuel cells and is expected to be used in fuel cells in the future.
Comment