Recently, the team of Academician Qiao Shi Zhang from the University of Adelaide in Australia has successfully enabled lithium-sulfur batteries to complete a full charge or discharge cycle within 5 minutes, addressing a key bottleneck issue for lithium-sulfur batteries.
Specifically, they took a series of carbon-based transition metal catalysts as examples and, based on Le Chatelier's principle, proposed for the first time the kinetic trend of the sulfur reduction reaction (SRR).
The content described by this trend is: the kinetics of the SRR will increase with the concentration of lithium polysulfide. In other words, there is a mathematical relationship between the two.
This trend indicates: by designing relevant catalysts, the concentration of lithium polysulfide can be increased, thereby enhancing the kinetics of the SRR.
In the study, the research team proved through synchrotron X-ray absorption spectroscopy measurements and molecular orbital theory calculations that the occupancy of the catalyst's anti-bonding orbital determines the concentration of polysulfides.Therefore, by adjusting the orbital occupancy of the catalyst, the concentration of lithium polysulfide can be enhanced, thereby improving the kinetics of the SRR reaction.
Advertisement
Utilizing the kinetic trends established in this study, the team designed a nano-composite CoZn/carbon catalyst and incorporated it into the cathode of lithium-sulfur batteries.
Under conditions of high sulfur loading (5mgcm-2), lean electrolyte (E/S=4.8), and high current density (8.0C), the lithium-sulfur battery achieved stable cycling with a power density as high as 26120W kgS-1, allowing the battery to complete charging and discharging within 5 minutes.
In summary, this work has introduced a novel concept, achieving both in-depth mechanism analysis and exceptionally excellent electrochemical performance.
It is anticipated that the high-power lithium-sulfur batteries developed in this study will have broad application prospects in portable electronic products and grid energy storage systems.Specifically, it can be used for a variety of devices, such as mobile phones, laptops, electric vehicles, large-scale power stations, and so on.
Due to the high power characteristics of this lithium-sulfur battery, it is particularly suitable for scenarios that require rapid charging and discharging. For example, when a mobile phone runs out of power, using this lithium-sulfur battery can fully charge the phone's battery in just a few minutes.
Furthermore, when an electric vehicle is about to run out of power, it can be driven into a charging station. At this point, the charging station can complete a full charge for electric vehicles equipped with this lithium-sulfur battery within a few minutes.
In general, the fast charging and discharging features of this lithium-sulfur battery will greatly facilitate people's daily lives.
In fact, the reason why the related research paper was published in a high-impact journal like Nature is that it solved a long-standing problem in the field.Before the start of this study, lithium-sulfur batteries had been developed for about 20 years, boasting a high energy density, 2-3 times higher than that of commercial lithium-ion batteries.
However, the power density of lithium-sulfur batteries remains low, meaning that the charging and discharging speed is slow. Throughout the 20 years of development of lithium-sulfur batteries, this issue has never been resolved.
Currently, it still takes several hours for a lithium-sulfur battery to complete a single charge and discharge cycle, which limits its application in scenarios requiring fast charging and discharging.
In 2021, Qiao Shizhen began to have his students research the issue of fast charging for lithium-sulfur batteries, that is, how to enhance the power density of the batteries.
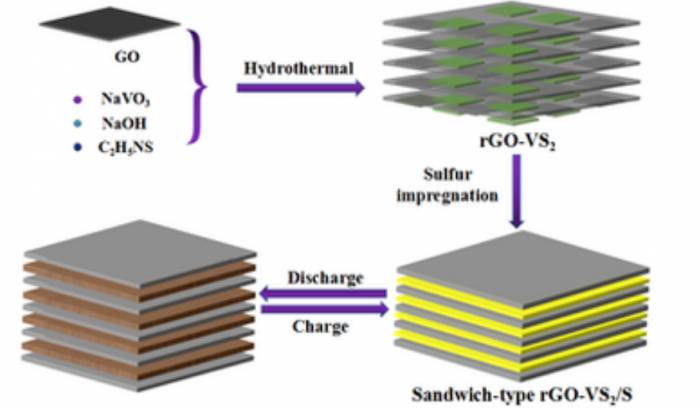
Due to the research group's solid foundation in catalyst design, they started to conceive the idea: could catalysts be used to achieve fast charging and discharging of lithium-sulfur batteries?Fundamentally, the slow charging and discharging of lithium-sulfur batteries stem from the slow conversion reactions of sulfur. The essence of a catalyst lies in its ability to reduce the activation energy of a reaction, thereby enhancing the reaction kinetics.
From this, it can be seen that the combination of lithium-sulfur batteries with catalysts can effectively address the bottleneck issue of slow charging and discharging rates in lithium-sulfur batteries.
Thus, they set this goal: to achieve fast charging and discharging of lithium-sulfur batteries using catalysts.
The charging and discharging rates of lithium-sulfur batteries are related to the kinetics of the sulfur reduction reaction. Therefore, the problem they face is: what parameter is the kinetics of the sulfur reduction reaction related to? And how to regulate and optimize the kinetics of sulfur reduction?
To solve the above problems, it is necessary to establish a kinetic trend of sulfur reduction.Concentration is the most fundamental parameter in describing kinetics. However, for the sulfur reduction reaction, it has been difficult to monitor the concentration changes of lithium polysulfide on the catalyst surface in situ in the past.
After long-term exploration, the team developed a set of in situ UV spectroscopy equipment, which realized the in situ monitoring of the law of lithium polysulfide concentration on the catalyst surface changing with voltage.
Taking a series of carbon-based transition metal catalysts such as Fe, Co, Ni, Cu, Zn, etc., as examples, they found that the kinetics of the sulfur reduction reaction is related to the concentration of lithium polysulfide on the catalyst surface, that is, the kinetics will increase with the increase of lithium polysulfide concentration.
This means that the kinetics of the sulfur reduction reaction need to be accelerated by increasing the concentration of lithium polysulfide on the catalyst surface.
So, what properties of the catalyst determine the concentration of lithium polysulfide? What kind of catalyst design is the most effective?By combining theoretical calculations with synchrotron radiation techniques, the research team has simultaneously verified this rule from both theoretical and experimental perspectives: that is, the electron occupancy of the catalyst's antibonding orbital determines the concentration of polysulfide lithium on the catalyst surface. Moreover, the eg/t2g values of different catalyst electronic orbitals are linearly correlated with the concentration of polysulfide lithium.
To this end, they designed a nanocomposite CoZn/carbon catalyst, which has higher eg/t2g values and a higher concentration of polysulfide lithium. As a bimetallic catalyst, its SRR performance is significantly better than that of other single-metal catalysts.
When used in lithium-sulfur batteries, the power density of the battery is greatly enhanced, allowing for rapid charging and discharging under conditions of high sulfur loading and lean electrolyte.
Ultimately, the related paper was published in Nature Nanotechnology (IF 38.3) with the title "Developing high-power Li||S batteries via transition metal/carbon nanocomposite electrocatalyst engineering."
Huan Li is the first author, and Qiao Shizhuang serves as the corresponding author[1].It is also reported that the research of this team is mainly based on "new materials," "new reactions," "new methods," and "new mechanisms."
In each research, Qiao Shizhang will guide students in the macro direction. When students get to a specific topic, he will ask: "What are the innovation and highlights of your topic? What is the difference between your work and the previously reported work? What is the practical significance of your work?"
"In general, our team's work is mainly based on the word 'new,' striving to innovate on past research, doing some different topics that make readers' eyes shine, while also improving performance and making progress towards practical applications," said Qiao Shizhang.
In fact, in the research of batteries, the team has also opened up many other directions, such as aqueous batteries, solid-state batteries, metal-sulfur batteries, and battery recycling.
Qiao Shizhang expressed that he believes the fast-charging concept and method proposed in this work can also be applied to other battery systems, and the team is currently conducting related research.In addition, the research group is also conducting related studies on the design of high-safety aqueous batteries, the development of solid-state electrolytes, the recycling of lithium-ion battery electrode materials, and the direction of high-energy-density and fast-charging metal-sulfur batteries.
Apart from battery-related research, the team has done a great deal of research work in electrocatalysis, photocatalysis, and theoretical calculations and machine learning.
Qiao Shizhuang said: "Our overall idea is to base on an innovative topic, combined with cutting-edge characterization methods, such as synchrotron radiation, in-situ spectroscopy, theoretical calculations, and machine learning, to develop new materials and new reactions, propose new methods, and explore new mechanisms of chemical reactions."
With a solid foundation in the field of electrocatalysis for many years, they have proposed new concepts such as electrorefining (E-Refinery), correlated single-atom catalysts (Correlated Single-atom Catalyst), the impact of surface microenvironment (Local Environment) on catalysis, and direct seawater electrolysis for hydrogen production (Direct Seawater Electrocatalysis for Hydrogen production), which are believed to provide certain guiding significance for the storage and conversion of electrochemical energy.
Comment